The Passive House concept represents a revolutionary approach to building design that prioritizes energy efficiency, comfort and sustainability. With growing concerns about climate change and energy consumption, Passive House principles are more important than ever. This architectural philosophy aims not only to reduce energy use, but also to create living spaces that enhance the well-being of building occupants.
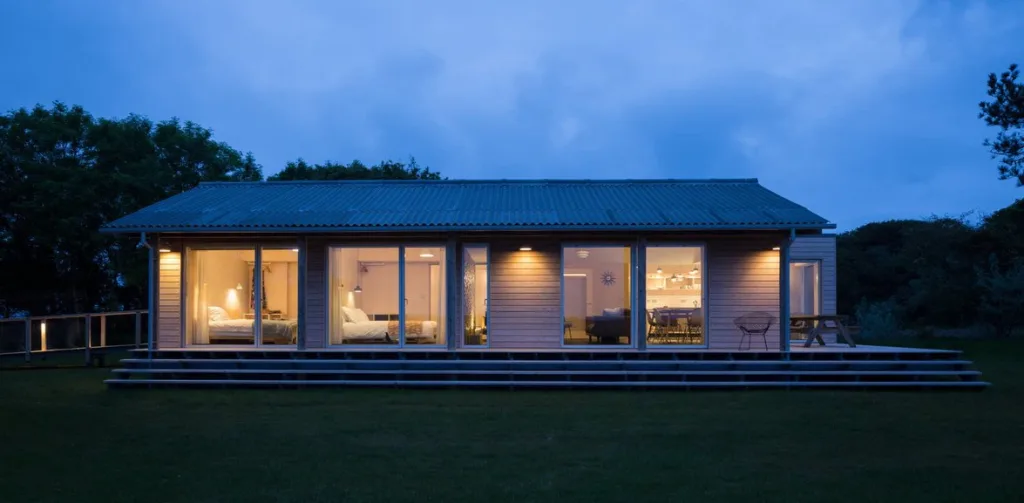
Passive House Definition
At its core, a Passive House is a building that meets certain energy efficiency criteria, minimizing its environmental impact while providing a high level of comfort. The term comes from the German word “Passivhaus” and refers to a rigorous standard for energy efficiency in a building. A Passive House typically requires very little energy for heating or cooling, allowing it to maintain a comfortable indoor climate year-round without conventional heating systems. This is achieved through careful design and the integration of high-performance materials and technologies.
Historical Background
The Passive House movement emerged in the late 20th century out of a desire to address the growing energy crisis and environmental degradation. The first Passive House was built in Germany in 1991 and served as a prototype for future designs. Over the years, the concept spread across Europe and eventually reached the United States and other parts of the world. This expansion was driven by advances in building technology and materials, as well as growing awareness of the effects of climate change. Today, the Passive House standard is internationally recognized and numerous buildings that adhere to its principles demonstrate its adaptability and effectiveness in various climates.
Basic Principles of Passive Design
The success of Passive House design is based on several key principles. First, a high level of insulation is essential. This prevents heat loss in winter and keeps the interior cool in summer. Secondly, the airtight construction minimizes air leaks that can lead to energy loss. Third, the design emphasizes the use of quality windows that provide natural light while reducing heat transfer. Furthermore, strategic ventilation systems ensure fresh air circulation without compromising energy efficiency. Finally, the orientation and layout of the building are critical; they take advantage of sunlight and optimize the use of sunlight for heating.
Benefits of Passive House Standards
The benefits of complying with Passive House standards are manifold. One of the most important benefits is the significant reduction in energy consumption. Buildings designed to this standard can use up to 90% less heating and cooling energy than conventional buildings. This not only means lower electricity bills for occupants, but also less pressure on local energy resources. Passive Houses also promote a healthier indoor environment by providing superior air quality through effective ventilation systems. This can lead to better health outcomes for occupants, making these homes not only energy efficient but also conducive to well-being.
Furthermore, Passive House designs contribute to reducing greenhouse gas emissions by utilizing sustainable practices and meet the urgent need for climate action. Many governments and organizations are promoting the construction of Passive Houses, recognizing their role in sustainable urban development.
Overview of the Role of Insulation
Insulation is one of the cornerstones of Passive House design. It acts as a thermal barrier that keeps the desired temperature inside the building constant, regardless of external conditions. Insulation in a Passive House is not only about the thickness of the material; it is also about its quality and placement. Seamless insulation without gaps is crucial, as even small openings can lead to significant energy loss.
Materials used for insulation can range from traditional options such as fiberglass to more innovative solutions such as cellulose or sheep wool. Each type offers unique benefits such as sustainability or improved thermal performance. In Passive House, the effectiveness of insulation ensures that heating and cooling systems, if any, operate minimally, ensuring that the building has a comfortable atmosphere throughout the year.
In summary, Passive House design principles underscore a commitment to energy efficiency, sustainability and occupant well-being. As we continue to meet the challenges posed by climate change, these principles provide a roadmap for creating buildings that are not only comfortable, but also responsible stewards of our planet.
Types of Insulation Materials
Insulation is a crucial component in building construction and renovation, providing thermal resistance that helps maintain comfortable indoor temperatures and improves energy efficiency. The choice of insulation material can significantly affect a building’s energy consumption, comfort level and environmental impact. Let’s examine the various types of insulation materials, each with their own unique properties and applications.
Fiberglass Insulation
One of the most widely used materials, fiberglass insulation is composed of tiny glass fibers that trap air and create a barrier against heat flow. This material is usually available in batt or roll form, making it easy to install in walls, attics and floors. The effectiveness of fiberglass insulation is measured by its R-value, which indicates its resistance to heat transfer. A higher R-value means better insulation performance.
In terms of applications, fiberglass is preferred in residential construction due to its affordability and versatility. It can also be found in commercial buildings where it helps regulate temperature and reduce energy costs. However, while fiberglass insulation is fireproof and moisture resistant, it can pose health risks if inhaled during installation. Therefore, protective equipment is essential during use.
Foam Board Insulation
Foam board insulation is a rigid panel made of polystyrene, polyisocyanurate or polyurethane. Known for its high insulation value per inch, foam board is especially effective in areas where space is limited, such as foundation walls, exterior walls and roofs. The closed-cell structure of foam board helps to resist moisture, making it suitable for use in climates with significant humidity or precipitation.
In practice, foam board insulation is often used in both new construction and retrofit projects. Its lightweight construction and ease of installation allows builders to ensure a tight seal against air leaks, improving overall energy efficiency. In addition, foam board can be combined with other types of insulation for optimized performance, making it a versatile choice in modern architecture.
Cellulose Insulation
Cellulose insulation is a sustainable option made from recycled paper products, primarily newsprint. This material is treated with fire retardants and is usually blown into wall cavities or attics, filling voids and creating a seamless thermal barrier. One of the highlights of cellulose is its excellent sound dampening properties, which improve acoustic comfort in residential and commercial spaces.
Cellulose insulation is particularly attractive to environmentally conscious builders and homeowners due to its recycled content and lower carbon footprint. It is also effective at moisture control, which helps prevent mold and mildew growth. As energy efficiency becomes increasingly important, cellulose insulation is being adopted in various green building projects, contributing to sustainable construction practices.
Mineral Wool Insulation
Mineral wool, also known as rock wool or slag wool, is made from natural or recycled materials such as basalt rock or industrial by-products. This type of insulation is known for its fire resistant properties, sound absorption abilities and resistance to moisture. Mineral wool is available in batts, rolls or loose-fill forms, making it adaptable for different applications.
In the construction industry, mineral wool is often used in commercial buildings, especially in areas where fire safety is a priority, such as around electrical equipment or partitions between units. Its ability to withstand high temperatures and provide sound insulation makes it an excellent choice for multi-family housing and industrial facilities. In addition, mineral wool does not burn and does not support the growth of mold and mildew, which adds another layer of safety and durability.
Natural Insulation Materials
Natural insulation materials such as sheep wool, cotton, straw and hemp are gaining popularity due to their environmentally friendly properties. These materials are renewable, biodegradable and often have lower environmental impacts compared to synthetic options. For example, sheep wool not only provides effective thermal insulation, but also has the ability to absorb moisture and helps regulate indoor humidity levels.
The use of natural insulation is often found in sustainable building projects where reducing carbon footprints and promoting environmental responsibility are primary goals. These materials can be used in applications ranging from residential to commercial buildings and contribute to healthier indoor air quality. As awareness of the environmental impacts of construction grows, natural insulation materials are increasingly recognized as viable alternatives to conventional options.
As a result, insulation material selection is vital for energy efficiency, comfort and environmental impact. Understanding the unique characteristics and applications of each insulation type can guide builders and homeowners to make informed choices that align with their specific needs and values.
3. Insulation Performance Metrics
Insulation acts as a crucial barrier between the inside and outside of a building, significantly impacting energy efficiency, comfort and overall sustainability. Understanding the various performance metrics helps architects and builders make informed choices about insulation materials and techniques, ultimately leading to better designed structures. This section examines a few key metrics that define insulation performance, highlighting their importance and real-world impact.
R-Value Explained
The R-Value is a fundamental measure in the world of insulation, representing the material’s resistance to heat flow. The higher the R-Value, the better the effectiveness of the insulation in preventing heat transfer. It is very important to understand that R-Value varies between different materials and thicknesses, making it essential for architects to choose the right insulation for specific climates and building designs.
For example, in colder regions, higher R-Values are required to keep interiors warm and comfortable during the winter months. Conversely, in warmer climates, lower R-Values may be sufficient, as the primary goal is to prevent heat from entering the building. Real-world applications of R-Value can be seen in residential construction, where selecting the appropriate insulation materials such as fiberglass, spray foam or cellulose can significantly impact energy bills and comfort levels.
Furthermore, R-Value plays a critical role in energy code compliance. Many building codes require a minimum R-Value for insulation in various parts of a structure, ensuring that new buildings meet energy efficiency standards. Therefore, understanding R-Value not only influences design choices, but also aligns with sustainability goals.
Thermal Bridging Effects
Thermal bridging occurs when materials with high thermal conductivity, such as metal or concrete, create a path for heat to flow through the envelope of a building, bypassing insulation. This phenomenon can lead to significant energy loss, making it essential for architects and builders to consider thermal bridging when designing buildings.
For example, in a steel-framed building, steel components can divert heat away from insulated areas, creating cold spots and increasing heating and cooling costs. To reduce thermal bridging, designers often incorporate insulation strategies such as continuous insulation or thermal breaks that interrupt the heat flow path.
The effects of thermal bridging go beyond energy loss; it can also affect indoor comfort and lead to condensation issues, contributing to moisture problems and mold growth. By addressing thermal bridges at the design stage, architects can improve the overall performance and longevity of the building while ensuring occupant comfort.
Air Leakage and Insulation
Air leakage refers to the unwanted movement of air in and out of a building, which can significantly undermine the effectiveness of insulation. Gaps, cracks and poorly insulated areas can allow warm air to escape in winter and cold air in summer, leading to increased energy consumption and higher utility bills.
Effective insulation works best when paired with airtight construction. This means that architects and builders should focus not only on insulation materials, but also on the tightness of the building envelope. Techniques such as weather stripping, caulking and the use of advanced framing methods can minimize air leakage by increasing the airtightness of a structure.
Real-world examples highlight the importance of air leakage control. Many high-performance buildings, such as Passive Houses, emphasize airtight construction and achieve energy efficiency through meticulous attention to detail. By focusing on both insulation and air leakage, buildings can provide comfortable indoor environments while reducing their environmental impact.
Moisture Management in Insulation
Moisture management is a critical aspect of insulation performance that is often overlooked. When insulation becomes damp, its effectiveness is reduced, leading to potential structural damage and health risks due to mold growth. The challenge lies in balancing moisture control with insulation performance, especially in climates where humidity levels fluctuate.
Architects must consider the materials used, as some types of insulation, such as fiberglass, are more resistant to moisture than others, such as cellulose, which can absorb water. The application of vapor barriers and appropriate drainage systems can help manage moisture levels, ensuring that insulation remains dry and effective.
For example, in basements and crawl spaces, using closed-cell spray foam insulation can provide both thermal resistance and moisture control, preventing water ingress and maintaining energy efficiency. By integrating moisture management strategies into insulation design, buildings can achieve better durability and indoor air quality.
Life Cycle Assessment of Insulation Materials
Life cycle assessment (LCA) of insulation materials evaluates their environmental impact from production to disposal. This comprehensive approach enables architects to understand not only the performance of insulation, but also its sustainability. Factors such as resource extraction, production energy, transportation emissions and end-of-life disposal all contribute to the overall environmental footprint.
For example, some insulation materials, such as recycled denim or cellulose, can have lower embodied energy compared to traditional fiberglass or foam options. By choosing materials with better LCA results, architects can create healthier buildings and support sustainable practices at the same time.
Real-world applications of LCA in insulation selection can be seen in green building certifications such as LEED, which rewards projects for using sustainable materials with appropriate life cycle profiles. By prioritizing LCA in insulation selection, builders can contribute to a more sustainable future by ensuring that their designs are not only efficient but also environmentally sound.
As a result, understanding insulation performance metrics is vital to creating energy-efficient, sustainable buildings. By considering R-Value, thermal bridging, air leakage, moisture management and life cycle assessments, architects and builders can make informed decisions that lead to healthier, more comfortable living spaces.
4. Design for Effective Insulation
Designing for effective insulation is one of the cornerstones of sustainable architecture. It not only improves energy efficiency, but also contributes to the comfort and well-being of building occupants. Insulation acts as a barrier, minimizing heat transfer and maintaining indoor temperatures. This chapter looks at various aspects of insulation design and examines how careful choices can lead to more sustainable and comfortable buildings.
Building Envelope Considerations
The building envelope is the physical separator between the internal and external environments of a structure. It includes walls, roofs, windows and doors. When designing for effective insulation, the performance of the outer shell is crucial. A well-designed facade minimizes energy loss while allowing natural light and ventilation.
Key considerations also include the choice of materials. For example, using materials with high thermal mass, such as concrete or brick, can help stabilize indoor temperatures. Furthermore, the design should incorporate appropriate sealing techniques to prevent air leaks that often undermine insulation efforts. It is also important to understand the relationship between the façade and the environment; for example, strategic placement of windows can maximize solar gain in cold climates, while overhangs can provide shade in warmer regions.
Real-world applications of effective building envelope design can be seen in passive house projects around the world. These homes prioritize insulation and airtightness and often achieve significantly lower levels of energy consumption than conventional buildings.
Insulation Installation Strategies
Where and how insulation is placed within a building can greatly affect its overall effectiveness. Insulation can be placed in various areas such as walls, ceilings and floors. Location affects not only thermal performance but also humidity control and sound insulation.
For best performance, insulation should be installed seamlessly, avoiding gaps that can lead to thermal bridges – areas where heat can easily escape. For example, in wall assemblies, insulation can be placed between studs, but also consider external insulation that wraps around the entire structure. This approach reduces thermal bridges and improves energy efficiency.
In practical terms, many modern buildings use insulated concrete forms (ICFs) or structural insulated panels (SIPs). These systems provide a high level of insulation and are designed to create a continuous thermal barrier that simplifies the installation process and improves overall performance.
Choosing the Right Thickness
The thickness of insulation is a critical factor in determining its effectiveness. In general, thicker insulation provides better thermal resistance. However, the right thickness often depends on several factors, including climate, building design and local building codes.
In colder climates, more insulation thickness is typically required to maintain comfortable indoor temperatures during the winter months. In contrast, in milder climates, a medium thickness of insulation may be sufficient. It is also important to balance insulation thickness with space constraints; for example, in urban environments where square meters are limited, architects must find creative solutions to maximize insulation without compromising habitable space.
A practical example can be seen in retrofitting old buildings. In these cases, adding insulation to the attic or walls may require careful thickness considerations to maintain the structural integrity of the building while improving energy efficiency.
Integration with Other Building Systems
Effective insulation design does not exist in a vacuum; it must be integrated with other building systems such as heating, ventilation and air conditioning (HVAC). Poorly integrated systems can lead to inefficiencies and reduced comfort levels in a building.
For example, a well-insulated building can still experience energy loss if the HVAC system is not properly sized or if there are leaks in the ducts. Therefore, collaboration between insulation design and HVAC planning is essential. Mechanical systems should be designed to work in harmony with the building envelope and ensure efficient and uniform delivery of conditioned air throughout the space.
Real-world examples of integrated systems can be found in modern green buildings that utilize advanced technologies such as heat recovery ventilators. These systems not only improve indoor air quality, but also work synergistically with insulation to reduce overall energy consumption.
Local Climate Assessments
Finally, local climate plays an important role in insulation design. The effectiveness of insulation materials and strategies can vary significantly depending on whether a building is located in a warm, humid climate or a cold, dry region. Understanding these local conditions is crucial for making informed decisions about insulation.
For example, in areas of high humidity, it is vital to choose insulation materials that resist moisture accumulation and prevent mold growth and structural damage. Conversely, in arid climates, the focus can be on maximizing thermal mass to absorb heat during the day and release it at night.
Architects and builders often conduct climate analysis to determine the most effective insulation strategies for their projects. This approach ensures that buildings are not only energy efficient, but also durable and comfortable in their environments. For example, in hot climates, the use of reflective roofing materials can significantly improve energy efficiency by reducing the heat absorbed into the building.
In summary, effective insulation design is a multi-faceted endeavor that requires careful consideration of materials, layout, thickness and integration with other systems while taking into account local climatic conditions. By adopting these principles, architects can create buildings that are not only energy efficient, but also improve the quality of life of the occupants.
5. Case Studies of Successful Passive Houses
Passive houses represent a groundbreaking approach to building design that prioritizes energy efficiency, comfort and sustainability. These structures use innovative techniques to maintain a stable indoor climate without relying heavily on conventional heating and cooling systems. The following case studies highlight various successful applications of passive house principles in different settings and illustrate how these concepts can be effectively applied in real world scenarios.
Example 1: Residential Passive House
A remarkable example of a residential passive house is located in Freiburg, Germany. This house exemplifies how thoughtful design can achieve significant energy savings while providing a comfortable living environment. The house has thick, insulated walls that minimize heat loss, triple-glazed windows that maximize sunlight while reducing glare, and a heat recovery ventilation system that provides a continuous circulation of fresh air without losing warmth.
The layout of the house is also optimized for passive solar gain. Large south-facing windows allow sunlight to fill the living spaces, naturally heating them throughout the day. The design incorporates overhangs to block excessive summer heat, ensuring that the indoor climate remains pleasant year-round. This house not only showcases the aesthetic possibilities of passive house design, but also serves as an inspiring model for homeowners looking to reduce their carbon footprint.
Example 2: Commercial Passive Building
The Sustainable Landscape Center in Pittsburgh, Pennsylvania, is a pioneering passive building in the field of commercial architecture. Housing offices and educational spaces, it is designed to achieve net zero energy use. Its design integrates renewable energy sources such as natural ventilation, thermal mass and solar panels.
The orientation of the building and window placement have been meticulously planned to optimize energy efficiency. Large windows invite natural light, reducing the need for artificial lighting throughout the day, while operable windows allow for cross ventilation during the warmer months. The use of local materials not only supports the regional economy but also reduces transportation emissions, making this commercial passive building a testament to sustainable practices in the corporate world.
Example 3: Passive House Retrofit
Retrofitting old buildings to meet passive house standards is an increasingly popular approach, and one striking example is the renovation of a century-old house in Toronto, Canada. This project involved improving insulation, replacing old windows with high-performance models and adding energy-efficient heating systems.
The transformation of this house demonstrates that passive house principles can be applied to existing structures, breathing new life into them while significantly reducing energy consumption. The project not only increased the comfort and aesthetic appeal of the house, but also reduced its environmental impact. This retrofit serves as a beacon for both homeowners and architects, proving that sustainability can be achieved even in older buildings.
Lessons Learned from Each Case
Each of these case studies offers valuable insights into the application of passive house principles. From the residential example in Freiburg, we learn that paying attention to orientation and solar gain can improve both comfort and energy efficiency. The commercial building in Pittsburgh shows us how businesses can lead the way in sustainable practices, teaching us the importance of integrating renewable energy sources and local materials.
The retrofit project in Toronto promotes sustainability in renovation, emphasizing that even old buildings can be transformed into energy-efficient homes. The common thread between these examples is the importance of holistic design that considers everything from orientation and materials to systems and user behavior.
Future Trends in Passive House Design
Looking ahead, the future of passive house design is bright and full of innovative possibilities. As technology advances, we can expect new materials and techniques that further improve energy efficiency. Smart home technologies will likely play an important role, allowing homeowners to monitor and adjust their energy use in real time.
Furthermore, urban planners are beginning to realize the potential of passive design in larger developments, leading to the creation of passive house neighborhoods that promote community living while minimizing environmental impact. More and more emphasis is being placed on entire ecosystems of sustainable living, not just individual buildings.
As the world increasingly prioritizes sustainability, passive houses are likely to become more common and influence building codes and standards globally. This evolution means a collective movement towards a more sustainable future where energy efficiency is not just an option, but a fundamental aspect of architecture.
6. Conclusion and Future Directions
Summary of Key Points
As we conclude our coverage of insulation in architecture, it is important to reflect on the key concepts we have covered. Insulation acts as a critical barrier in buildings, helping to regulate temperature and improve energy efficiency. By reducing heat loss in winter and keeping interiors cool in summer, effective insulation contributes significantly to occupant comfort while lowering energy bills. We saw how materials such as fiberglass, foam and cellulose each bring unique properties to the table and affect not only thermal performance but also environmental impact.
Furthermore, the discussion highlighted the growing importance of passive design strategies in modern architecture. Prioritizing natural heating, cooling and lighting, these approaches rely heavily on high-quality insulation to maximize their effectiveness. The integration of smart technologies and sustainable materials is pushing us towards greener building practices, demonstrating the ongoing evolution in this field
.
The Future of Insulation in Architecture
Looking ahead, the future of insulation in architecture looks promising but challenging. As climate change intensifies, the built environment must adapt to new environmental realities. This means developing insulation solutions that not only meet strict energy rules, but also contribute to the overall durability of buildings. Innovations in bio-based materials that are not only effective but also reduce the carbon footprint of construction are gaining traction.
Also on the horizon is the rise of smart insulation technologies. Imagine materials that can adjust their thermal properties in response to changing external conditions or actively monitor energy use to optimize performance. This level of adaptability could revolutionize the way we think about insulating our homes and buildings, making them smarter and more efficient than ever before.
Policy Implications for Passive Design
The role of policy in shaping the future of insulation and passive design cannot be ignored. Governments are increasingly recognizing the need for regulations that promote energy efficiency and sustainable building practices. Building codes are evolving to mandate higher insulation standards and encourage the incorporation of passive design principles.
In many regions, incentives for the use of renewable materials and technologies are being created, making it financially viable for both developers and homeowners to invest in better insulation. However, for these policies to be effective, there needs to be continuous education and awareness-raising among architects, builders and the public about the benefits of passive design. Only through a coordinated effort can we ensure that buildings are not just structures, but integral parts of a sustainable future.
Innovations in Insulation Technology
The landscape of insulation technology is rapidly changing with the emergence of groundbreaking innovations that promise to improve performance while minimizing environmental impact. One exciting development is the use of aerogel, a material known for its incredible insulating properties and lightweight construction. Originally developed for space applications, aerogel is now finding its way into residential and commercial buildings, providing superior thermal insulation in thinner profiles.
Another notable innovation is the integration of phase change materials (PCMs) into insulation systems. These materials can absorb, store and release thermal energy, thus helping to regulate indoor temperatures more effectively. By incorporating PCMs into the building envelope, architects can create spaces that remain comfortable with less reliance on heating and cooling systems.
Furthermore, the focus on recyclability and sustainability has led to the emergence of insulation products made from recycled materials such as denim or agricultural waste. These options not only reduce waste but also offer effective thermal resistance, demonstrating that innovation in insulation can go hand in hand with environmental responsibility.
Call to Action for Sustainable Building Practices
As we conclude our exploration of insulation and its important role in architecture, there is a clear call to action for all stakeholders involved in the built environment. Architects, builders, policymakers and consumers must work collaboratively to adopt sustainable building practices that prioritize energy efficiency and environmental stewardship.
Investing in high-quality insulation is not just a choice; it is a commitment to creating a healthier planet. By choosing sustainable materials and advocating for policies that support passive design, together we can contribute to a future where buildings are not only energy efficient but also resilient to the challenges of climate change.
Let’s support innovations in insulation technology, support regulations that promote sustainability, and educate ourselves and others about the importance of these practices. Together, we can build a world where architecture not only serves its inhabitants, but also respects and nurtures the environment we all share.